A longstanding issue in evolutionary paleontology has been how to interpret the morphological gaps between various lineages in the fossil records. Apart from Cuvierian catastrophism and de Vriesian saltationism, the following four views are held, which are neither inconsistent with one another, nor conflict with the accepted mechanisms of microevolution.
1) Superficial morphological discontinuity due to the gap of sedimentation.-This represents the traditional reasoning of stratigraphers and paleontologists, and is said by some punctuationists to have been derived from the notion of "phyletic gradualism". Brinkmann's (1929) interpretation on the morphological break in a lineage of Kosmoceras (recently restudied by Raup and Crick (1981, 1982) from the statistical viewpoint) and Newell's (1956, fig. 3) generalized diagram, in which a lineage with continuous and unidirectional change of morphology is interrupted by some gaps of sedimentation, are typical examples of this view.

2) Essential morphological discontinuity due to the inconstant rate of morphological change within a lineage.-If stepwise morphological change occurs within a lineage, it is assumed that evolutionary patterns generally become intermittent, since the process of rapid transformation can rarely be traced in the fossil records. This view was popularized by Simpson (1953), who considered that the origin of a higher taxon can often be sought in some rapid breakthrough of a threshold between different adaptive zones.
3) Essential morphological discontinuity due to allopatric speciation and subsequent migration.-This view is an expansion of Mayr's (1942, 1963) theory of geographic speciation-that is, a new species arises rapidly from a peripherally isolated small population. This model was, I think, substantially adopted by Simpson (1953) in his interpretation of the trilobite sequence in the Cambrian of Sweden, and recently much emphasized by Eldredge and Gould (1972) and others as an alternative to phyletic gradualism. In this model it is generally anticipated that rapid genetic change at speciation results in rapid morphological change and that ecologic replacement of an existing species by an immigrant new species results in a punctuated pattern in the fossil records.
4) Essential and intrinsic morphological discontinuity due to phenotypic substitution.-If a single or a few genetic factors strongly influence morphological difference in an evolving population, phenotypic substitution (or the process from the introduction to the fixation of a mutant) would result in essential morphological discontinuity (Hayami and Ozawa, 1975). This process is quite different from the saltationists' "systemic mutation" and "Grossmutation", but, whether adaptive or non-adaptive, is not inconsistent with the acknowledged mechanism of evolution.
Morphological discontinuity in the fossil records, I believe, may occur due to various
geological and biological causes such as these. Among others, sedimentary gaps may be ubiquitous geological phenomena, representing a quite extrinsic cause for morphological discontinuity of various scales, because they must occur regardless of evolutionary pattern. This notion is therefore, by no means the exclusive property of the gradualists. Pure gradualism may be better defined in this case as the notion that all the observed morphological gaps should be attributed to the incompleteness of fossil records. The three other possible causes are biologically more fundamental. The problem is their relative frequency and scale rather than their adequacy.
As was already discussed in the preceding chapter, the evolution of Cryptopecten seems to be characterized by the presence of a few well-established, long-ranging species and the sudden appearance of some short-ranging unsuccessful branches. Some phyletic (sometimes directional) change actually occurred in the lineage of C. vesiculosus and possibly also in other lineages, but the relatively conservative morphology of long-ranging species and the rareness of morphologically transitional fossil samples may be consistent with the punctuational model.
The proposal of the punctuational model of morphological change (EIdredge and Gould, 1972; Gould and EIdredge, 1977) had enormous impact on evolutionists and has recently developed into more comprehensive tlieories of macroevolution such as higherlevel selection and hierarchical concept of evolution (Stanley, 1979; Gould, 1982; etc.). As emphasized by some of these authors, this model, though potentially testable, has not yet been tested to satisfaction. To do so would require excellent material and patient field and laboratory work. Immediately after the first proposal of this model, many paleontologists attempted to test it on the basis of their own data; some were found to agree but others not. So far as I am aware, however, most of the evidence cited to support this model is still circumstantial. On the other hand, gradual change of morphological unit characters was demonstrated in some lineages of animals by recent morphometric studies at the population level. Though well-documented examples of long-ranging, gradual transformation seem to be restricted to some protozoan lineages (Ozawa, 1975; Kellogg, 1975; Malmgren and Kennett, 1981; etc.), it is certain that morphology often changes gradually with or without a definite trend in metazoan lineages. Phyletic size increase is common in various lineages of Jurassic Bivalvia in western Europe (Hallam, 1975). Phyletic decreases in shell size and number of radial ribs are well recognized in the stock of C. vesiculosus, offering another example of gradual change within a lineage.
Nevertheless, I am unable to agree with the theory of phyletic gradualism. The actual macroscopic pattern of morphological change must, I think, resemble the punctuational model. Paleontologists are want to give a decision in favor of the party possessing the more plentiful circumstantial evidence. In this case, however, the debate between the gradualists and the punctuationists should not be decided so easily, for since geographic (allopatric) speciation, as considered by Mayr, probably occurs in a geological instant, and the process is bound to be less frequently recorded in fossil sequences than that of phyletic evolution.
Very recently, Williamson (1981) reported an interesting sequence of molluscan faunas in Late Cenozoic lacustrine deposits in the Turkana Basin of northern Kenya, discussing this current problem on the basis of actual paleontological data. Though the full account would be desirably published in some separate article, his material probably represents the best documented process of molluscan speciation that we have. According to him, in the lacustrine deposits, there are about ten long-ranging lineages of gastropods and bivalves with various reproductive strategies, which are morphologically very conservative throughout the sequence. At two definite horizons, however, new forms (possibly new species) arose simultaneously through rapid morphological change from many of these stocks, but they survived only temporarily to be suddenly replaced by the populations of conservative morphology without any intermediates. These incipient forms, which were said to have arisen from isolated populations by lacustrine regressions, seem to have become extinct when their parental species came back to the same lake through succeeding transgressions. Williamson interpreted these evolutionary patterns as being in evident conformity with Mayr's "founder principle" as well as with Eldredge and Gould's "punctuated equilibria". However, it is generally difficult to reconstruct the process of speciation in the case of marine molluscs from the data in this kind of local sequence.
My own experience leads me to feel that the theory of punctuated evolution is valid. Many morphological gaps may be caused by such episodic events as speciation, migration and extinction. I feel that Gould and Eldredge's (1977) claim, "Stasis is data", deserves serious consideration by evolutionary paleontologists. In current evolutionary studies, as they pointed out, the dynamic nature of a lineage is apt to be unfairly emphasized at the expense of the static. Thus although I concluded in the present study the directional shift of three characters, i.e., shell size, average number of radial ribs, and relative frequency of mutant phenotype, in the lineage of C. vesiculosus, but significant evolutionary change could not be detected for many other morphometric characters. It is tentatively supposed that these "static" characters are greatly influenced, not only by genetic causes, but also by environmental factors, and that the wide range of variation (or the too small sample size) makes it difficult to recognize chronological change. At the same time, however, I cannot necessarily deny the possibility that they are truly static.
Aside from the discrete variation of sculpture in C. vesiculosus, the net amount of observed morphological change within each lineage of Cryptopecten is, in every way, much less significant in comparison with the morphological difference between distinct lineages, The results of this study also suggest the possibility that many taxa (either species or subspecies) may arise from well-established stocks through isolation. Some of these isolated populations may grow into distinct species, and others may become extinct or be absorbed in the parental population in the main distribution. I believe that the three short-ranging taxa of Cryptopecten, i. e., C. vesiculosus makiyamai, C. nux sematensis and C. spinosus, represent only a fraction of the isolation and speciation that actually occurred. Speciation may be a ubiquitous event, many other unsuccessful, dead-end branches having been lost from the fossil records. This is why, in spite of the recognized gradual morphological change in the lineage of C. vesiculosus, I prefer the punctuated model.
A few reservations remain, however. Firstly, the current punctuationists' reason for attributing the cause of rapid morphological change almost exclusively to speciation still fails to completely convince. May not rapid morphological change also occur through the breakthrough of adaptive threshold or through bottle-neck effect within a single lineage? Secondly, the view that reproductive isolation is always accompanied by significant
morphological shift (or vice versa) may be too straightforward, given the many phenomena in nature (e. g., sibling species and polytypic species) where this is not the case.
Finally, I will discuss the significance of phenotypic substitution in the pattern and process of evolution. Though well-documented examples are still scarce, this is the most intrinsic cause of punctuated pattern. Frequency is not a problem, because polymorphism is a widespread phenomenon in living organisms. Questions about its role in macroevolution concentrate on scale:-how can significant morphological change be accomplished by a single mutation. Gould and Eldredge (1977) regarded my Cryptopecten data of phenotypic substitution (Hayami, 1973) as being too microscopic to test their punctuational model. Certainly, phenotypic substitution is not a case of transspecific evolution but represents a peculiar example of phyletic evolution.
It has been generally supposed that a new species or a higher taxon cannot arise from a single gene or chromosomal mutation. Recent advancement in the studies of molecular biology, however, seems to suggest that significant evolutionary change may occur within a lineage by the mutation of a "regulatory gene" and its fixation in the population. With the diffusion of modern synthetic evolutionary theory, Goldschmidt's (1940) anticipation of a "hopeful monster" was nearly abandoned for its too extreme notion of the instantaneous establishment of a new higher taxon. Nevertheless, polyploidy is still credible as a possible cause of instantaneous speciation. According to Menzel (1968) and Patterson (1969), chromosome numbers are relatively stable among the species within a bivalve family, but considerably variable among different families. Though cytological studies of bivalves seem to lag far much behind those of gastropods (especially nonmarine species), the role of polyploidy and other chromosomal change in macroevolution (especially the punctuated pattern of morphological change) is an interesting theme in evolutionary paleontology and should be investigated. Moreover, as was discussed by Stanley (1979) and others, future studies of "regulatory genes" may result in the resurrection of the macrogenesis theory, though in a form quite different from Goldschmidt's concept.
The observed morphological gap between the two phenotypes of C. vesiculosus is so clear that I wonder why few previous taxonomists regarded them as specifically distinct. If only the features of surface sculpture were taken into consideration, the difference between the two phenotypes would appear to be more significant than that between different species. I also expect that some related but morphologically distinct nominal molluscan "taxa" will prove to constitute discontinuous variation within an interbreeding population. The relation between Volachlamys hirasei and V. awajiensis may prove to be such a case. Evaluation of the role of phenotypic substitution depends upon careful studies of the relation between phena and taxa in living and fossil populations.
As was diagramatically shown in Fig. 24 and will be described in the chapter on systematics, in Cryptopecten there are two alternative modes in the disposition of imbricated scales on the sides of radial ribs. One is the alternate type, as seen in all the observed individuals of C. bullatus (Plate 2, Fig. 3e: Plate 11, Figs. 3a, b), C. nux (Plate 2, Fig. 4g) and C. phrygium (Plate 9, Figs. 6a, b, 7a, b). The other is the opposite type, as seen in the fresh specimens of C. yanagawaensis (Masuda, 1958, pl. 27b, fig. 8), C. vesiculosus (Plate 5, Figs. 1e, f, 2c, d, 3e, f; Plate 11, Figs. 1a, b, 2a, b) and C. spinosus (Plate 8, Fig. 5; Plate 12, Fig. 5). Because the relation between the two species groups has not yet been clarified on the basis of concrete evidence, it is now difficult to say which type is the more primitive. Aside from the phylogenetic relation, gradual dislocation of scales from one type to the other is rather unlikely, because the periodicity is regular in both types. The ultimate origin of this change, therefore, should be sought in a single mutation; that is to say, one type may have arisen from the other through phenotypic substitution. If the phenotypic substitution were rapid, the process could hardly be expected to be recognizable in the fossil records. It would likely appear to be a case of an ancestral "species" suddenly becoming extinct and a descendant "species" suddenly appearing, as was discussed by Hayami and Ozawa (1975). Species and higher taxa are often discriminated on the basis of alternative character, which may be possibly switched by a rather simple genetic cord. If the suggested existence of "regulatory genes" is taken into consideration, the role of phenotypic substitution occurring in a geological instant, particularly as the ultimate origin of a new alternative taxonomic character, should not be underestimated in the studies of macroevolution.

 Explanation of Plate 2
(All figures × 2, unless otherwise stated)
Figs. 1-3. Cryptopecten bullatus (Dautzenberg and Bavay)
Fig. 1. Right valve (UMUT CM16001a), sample Kk (5), Late Pleistocene Wan Formation at Kikai Island; 1a: external view, 1b: internal view.
Fig. 2. Left valve (UMUT CM16001b), the same sample; 2a: external view, 2b: internal view.
Fig. 3. Living conjoined valves (UMUT RM16007a), sample Ks (B), mouth of Kii Strait; 3a: external view of right valve, 3b: external view of left valve, 3c: anterior view, 3d: dorsal view, 3e: ornament on ventral area of right valve showing alternate disposition of imbricated scales (×5, with whitening), 3f: byssal wing (×5, with whitening).
Fig. 4. Cryptopecten nux nux (Reeve)
Fig. 4. Living conjoined valves (UMUT RM16020), sample An (N), mouth of Ise Bay; 4a: external view of right valve, 4b: internal view of right valve, 4c: external view of left valve, 4d: internal view of left valve, 4e: anterior view, 4f: dorsal view, 4g: ornament on ventral area of right valve showing alternate disposition of almost exfoliated imbricated scales (× 5, with whitening), 4h: byssal wing (×5, with whitening) |
 Explanation of Plate 3
(All figures ×2, unless otherwise stated)
Figs. 1-2. Cryptopecten nux nux (Reeve)
Fig. 1. Right valve (UMUT CM16015c), sample Kk (N), Late Pleistocene Wan Formation at Kikai Island; 1a: external view, 1b: internal view, lc: anterior view, ld: dorsal view.
Fig. 2. Left valve (UMUT CM16015d), the same sample; 2a: external view, 2b: internal view, 2c: anterior view, 2d: dorsal view.
Fig, 3-4: Cryptopecten nux sematensis (Oyama)
Fig. 3. Right valve (UMUT CM21561), Holotype, Yokoyama's specimen (1922, pl. 15, fig. 1), "Shito" (probably identical with the locality of sample Sm (N)); 3a: external view, 3b: internal view, 3c: anterior view, 3d: dorsal view.
Fig. 4. Left valve (UMUT CM16180a), sample Sm (N), Late Pleistocene Yabu Formation at Ochishimoshinden; 4a: external view, 4b: internal view, 4c: anterior view, 4d: dorsal view.
Fig. 5. Cryptopecten vesiculosus vesiculosus (Dunker)
Fig. 5. Population sample (UMUT RM16138), sample Is, mouth of Ise Bay; Left: individuals belonging to Phenotype R, Right: individuals belonging to Phenotype Q. (×0.8) |
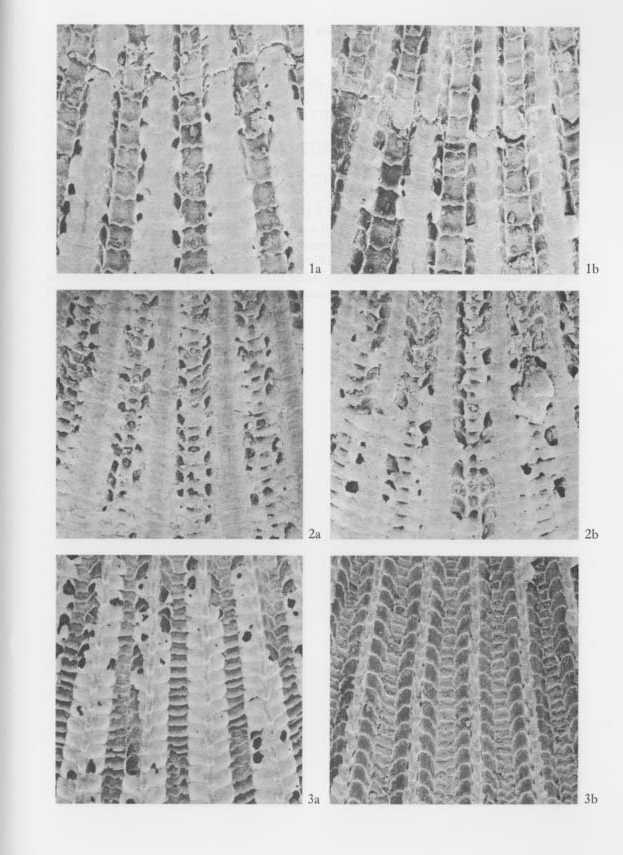 Explanation of Plate 11
(SEM photographs, all figures × 29, 5 KV)
Figs. 1, 2. Cryptopecten vesiculosus vesiculosus (Dunker)
Fig. 1. Living conjoined valves (UMUT RM16059a), Phenotype Q,, the same specimen as Pl. 10, Fig. 1; 1a: middle part of disk of right valve, 1b: middle part of disk of left valve.
Fig. 2. Living conjoined valves (UMUT RM16059b), Phenotype R, the same specimen as Pl. 10, Fig. 2; 2a: middle part of disk of right valve, 2b: middle part of disk of left valve.
Fig. 3. Cryptopecten bullatus (Dautzenberg and Bavay)
Fig. 3. Living conjoined valves (UMUT RM16002a), the same specimen as Pl. 10, Fig. 3; 3a: middle part of disk of right valve, 3b: middle part of disk of left valve. |

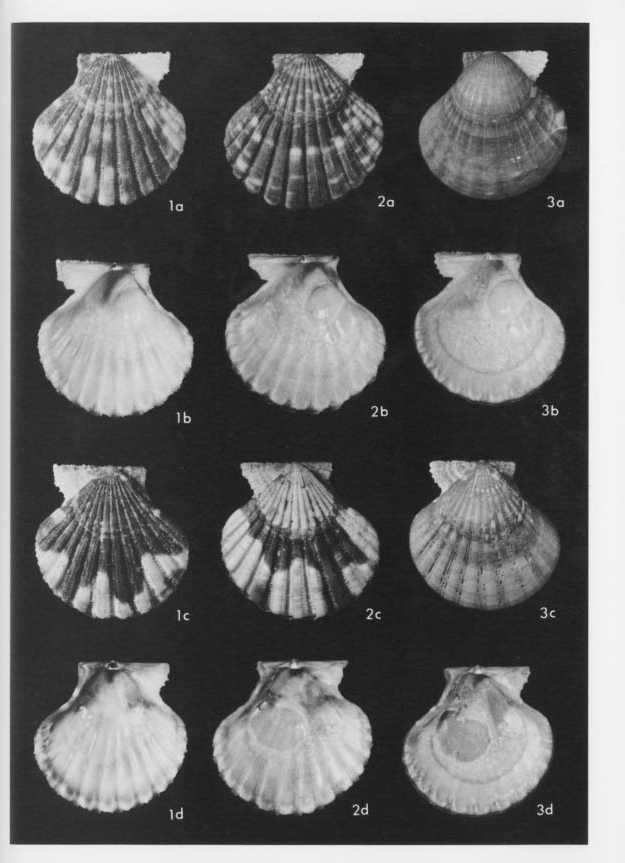 Explanation of Plate 4
(All figures ×l.5)
Figs. 1-3. Cryptopecten vesiculosus vesiculosus (Dunker)
Fig. 1. Living conjoined valves (UMUT RM16138b), Phenotype Q (rough subphcnotype), sample Is, mouth of Ise Bay; 1a: external view of right valve, 1b: internal view of right valve, 1c: external view of left valve, 1d: internal view of left valve.
Fig. 2. Living conjoined valves (UMUT RM16138c), Phenotype Q (smooth subphenotype), the same sample; 2a: external view of right valve, 2b: internal view of right valve, 2c: external view of left valve, 2d: internal view of left valve.
Fig. 3. Living conjoined valves (UMUT RM16138d), Phenotype R, the same sample; 3a: external view of right valve, 3b: internal view of right valve, 3c: external view of left valve, 3d: internal view of left valve. |
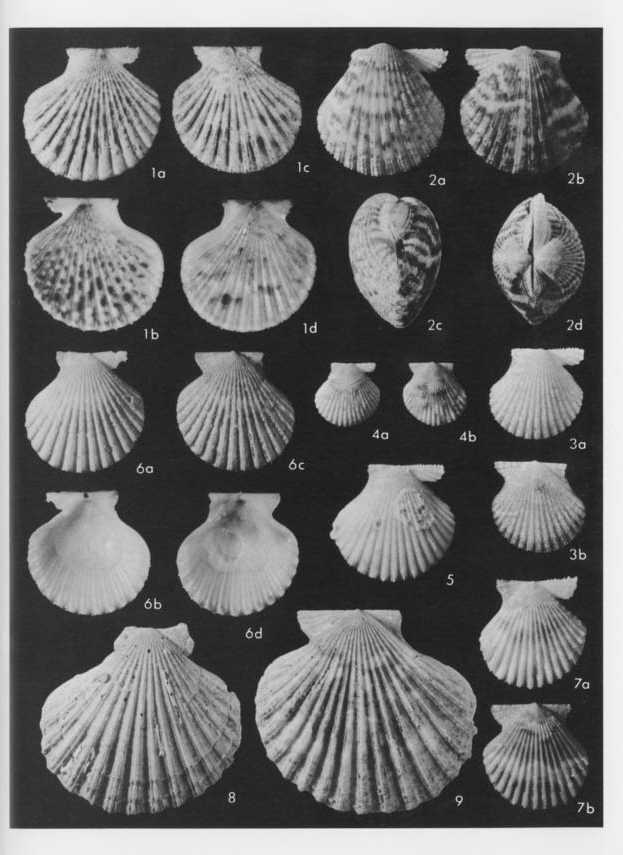 Explanation of Plate 9
Fig. 1. Cryptopecten bullatus (Dautzenberg and Bavay)
Fig. 1. Living conjoined valves (USNM 173194), Holotype of Cryptopecten alli Dall, Bartsch and Rehder, 1938; × 1.5; off south coast of Oahu, Hawaii; 1a: external view of right valve, 1b: internal view of right valve, 1c: external view of left valve, 1d: internal view of left valve.
Figs. 2. Cryptopecten nux nux (Reeve)
Fig. 2. Living conjoined valves (USNM 764157); × 2; Hervey Bay, Queensland; 2a: external view of right valve, 2b: external view of left valve, 2c: anterior view, 2d: dorsal view.
Fig. 3. Living conjoined valves (USNM 764164); × 2; Jolo, Philippines; 3a: external view of right valve, 3b: external view of left valve.
Fig. 4. Living conjoined valves (USNM 764160); × 2; Itoman, Okinawa, Japan; 4a: external view of right valve, 4b: external view of left valve.
Fig. 5. Right valve (USNM 764165); ×2; Al Ghardaqa, Egypt.
Figs. 6-9. Cryptopecten phrygium (Dall)
Fig. 6. Living conjoined valves (USNM 62352); × 1.5; Mexican Gulf; 6a: external view of right valve, 6b: internal view of right valve, 6c: external view of left valve, 6d: internal view of left valve.
Fig. 7. Living conjoined valves (USNM 764544); × 2; off Hillsboro, Florida; 7a: external view of right valve, 7b: external view of left valve.
Fig. 8. Right valve (USNM 694425); × 1.2; Mexican Gulf.
Fig. 9. Left valve (USNM 764542); × 1.2; off southwest Louisiana, Mexican Gulf. |
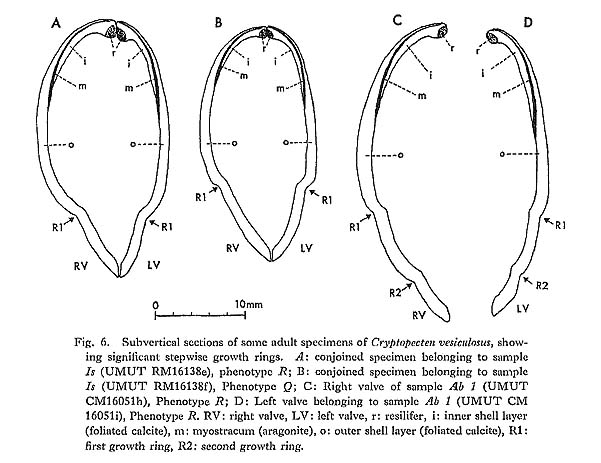
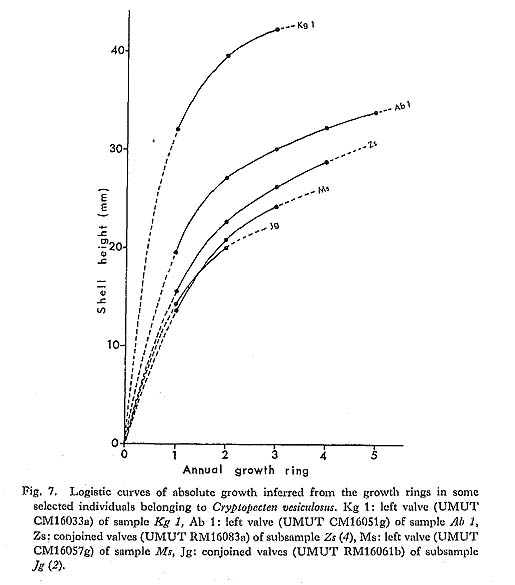

 Explanation of Plate 5
(× 1.5 for Figs. 1a-c, 2a, b, 3a-c, ×4.5 for Figs. 1d-f, 2c, d, 3d-f)
Figs. 1-3. Cryptopecten vesiculosus vesiculosus (Dunker)
Fig. 1. Living conjoined valves (UMUT RM16138b), the same specimen as Pl. 4, Fig. 1; la: anterior view, lb: dorsal view, lc: ventral view, ld: byssal wing (with whitening), le: ventral area of right valve (with whitening), lf: ventral area of left valve (with whitening).
Fig. 2. Living conjoined valves (UMUT RM16138c), the same specimen as Pl. 4, Fig. 2; 2a: anterior view, 2b: dorsal view, 2c: ventral area of right valve (with whitening), 2d: ventral area of left valve (with whitening).
Fig. 3. Living conjoined valves (UMUT RM16138d), the same specimen as Pl. 4, Fig. 3; 3a: anterior view, 3b: dorsal view, 3c: ventral view, 3d: byssal wing (with whitening), 3e: ventral area of right valve (with whitening), 3f: ventral area of left valve (with whitening). |
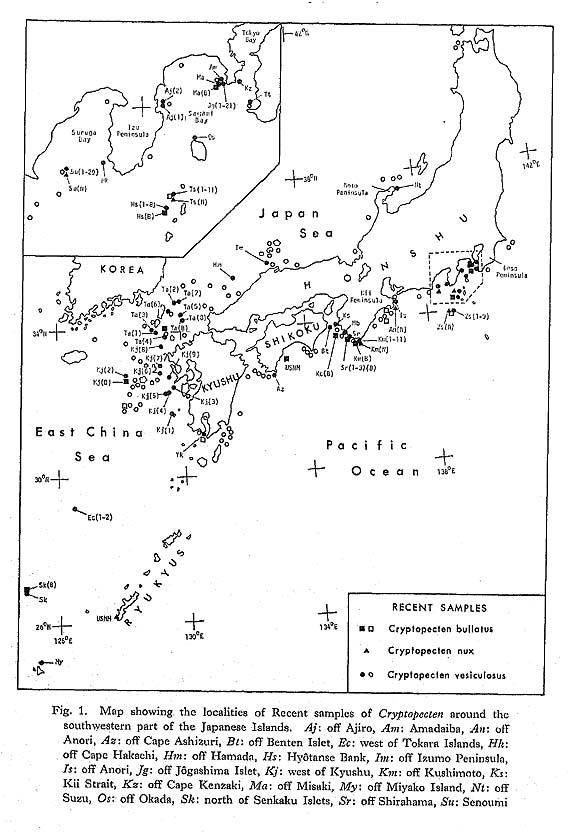
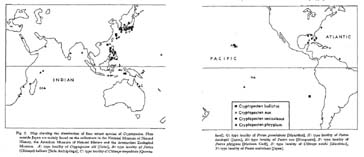
Fig. 2. Map showing the distribution of four extant species of Cryptopecten. Plots outside Japan are mainly based on the collections in the National Museum of Natural History, the American Museum of Natural History and the Amsterdam Zoological Museum. A: type locality of Cryptopecten alli [Oahu], B: type locality of Pecten (Chlamys) bullatus [Sulu Archipelago], C: type locality of Chlamys corymbiata [Queens-land], G: type locality of Pecten guendolenae [Mauritius], H: type locality of Pecten hastingsii [Japan], N: type locality of Pecten nux [Marquesas], P: type locality of Pecten phrygium [Mexican Gulf], S: type locality of Chlamys smithi [Mauritius], V: type locality of Pecten vesiculosus [Japan].
|
 Explanation of Plate 8
(All figures × 1.5, unless otherwise stated)
Figs. 1-5. Cryptopecten spinosus sp. nov.
Fig. 1. Right valve (UMUT CM16170d), Paratype, sample Kk (S), Late Pleistocene Wan Formation at Kamikatetsu, Kikai Island; 1a: external view, 1b: internal view.
Fig. 2. Left valve (UMUT CM16170c), Paratype, the same sample; 2a: external view, 2b: internal view, 2c: dorsal view, 2d: anterior view.
Fig. 3. Right valve (UMUT CM16170e), Holotype, the same sample; 3a: external view, 3b: internal view, 3c: dorsal view, 3d: anterior view, 3e: byssal wing. ( × 4)
Fig. 4. Left valve (UMUT CM16170f), Paratype, the same sample; 4a: external view, 4b: internal view.
Fig, 5. Ventral ar ea of right valve (UMUT CM16170g), Paratype, the same sample. ( × 4)
Fig. 6-9. Cryptopecten yanagawaensis (Nomura and Zinbo)
Fig. 6. Right valve (UMUT CM16171a), sample Mn (Y), Middle Miocene Moniwa Formation at Junishin near Natori.
Fig. 7. Left valve (UMUT CM16171b), the same sample.
Fig. 8. Left valve (UMUT CM16171c), the same sample.
Fig. 9. Right valve (UMUT CM16171d), the same sample. |

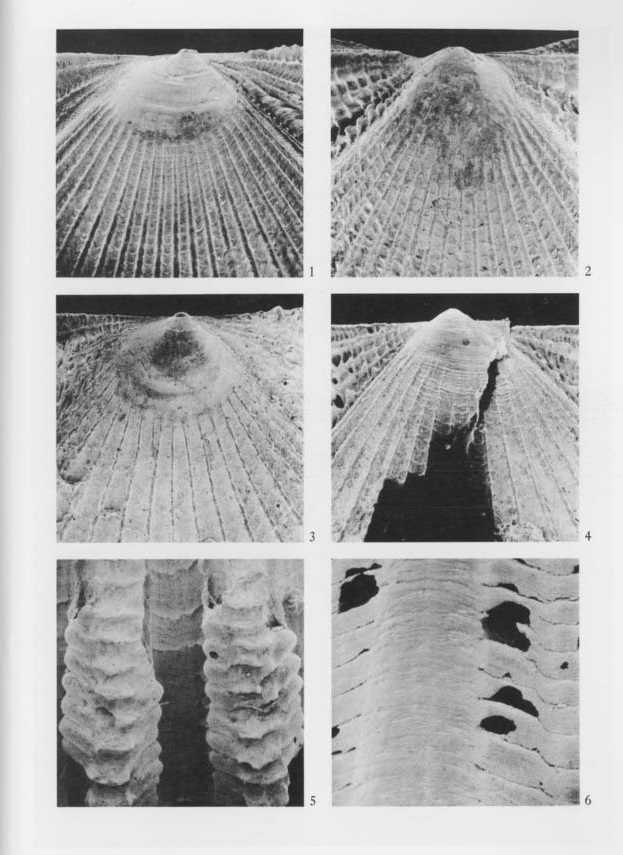 Explanation of Plate 12
(SEM photographs, all figures ×29, 5 KV)
Figs. 1, 2. Cryptopecten nux nux (Reeve)
Fig. 1. Umbonal area of right valve (UMUT CM16015a), sample Kk (N), Late Pleistocene Wan Formation at Kikai Island.
Fig. 2. Umbonal area of left valve (UMUT CM1601Sb), the same sample.
Figs. 3-5. Cryptopecten spinosus sp. nov.
Fig. 3. Umbonal area of right valve (UMUT CM16170a), sample Kk (S), Late Pleistocene Wan Formation at Kikai Island.
Fig. 4. Umbonal area of left valve (UMUT CM16170b), the same sample.
Fig. 5. Radial ribs on ventral area of right valve (UMUT CM16170a), the same specimen as Pl. 12, Fig. 3, showing oppositely disposed imbricated scales.
Fig. 6. Cryptopecten vesiculosus vesiculosus (Dunker)
Fig. 6. Radial rib on middle-ventral area of adult left valve (UMUT RM16061a), Phenotype R, sample Jg (2), off Jôgashima Islet, Sagami Bay, showing oppositely disposed imbricated scales. |
|