As was fully discussed and enumerated by Mayr (1963, pp. 138-163; 1969, pp. 144-163), individual variation within a single interbreeding population may be caused by various genetic and nongenetic factors. Because environmentally induced morphological modification has almost nothing to do with evolutionary change, causal evaluation of variation, though not necessarily easy in fossil and marine molluscs, is of primary im-portance for evolutionary studies of this kind.
Individual variation of morphological characters are classifiable, though only for con-venience, into two types: continuous and discontinuous. Various morphometric (including meristic) characters such as body size at a definite growth stage, form ratios between two linear measurements, angles, and number of serially arranged organs commonly vary continuously, and usually show histograms resembling normal frequency distribution. This continuously varied unit character is generally regarded as being controlled by a large number of genetic and environmental factors. Discontinuous variation, on the other hand, is thought to be controlled by a single or a few genetic or nongenetic factor(s), Both types of individual variation are seen in each large population of Cryptopecten.
Since Cryptopecten bullatus, Cryptopecten nux and extinct species of this genus are represented by relatively small samples in the present collection, the detailed examination of intrapopulational variation is for the most part restricted to several large samples of C. vesiculosus. The size of some of the samples precluded measurement of all the specimens, so 50 right and 50 left (or 50 conjoined) valves were randomly selected. In Middle Pleistocene and later samples two discrete phenotypes are distinguishable by the sculpture of the disk, and they were treated separately. Computations for statistics were made on these reduced samples excluding some broken valves which were inadequate for measurement. Meristic variation was examined on these and several more samples. Phenotypic frequency of dimorphism however, was examined on the basis of all indi-viduals in every fossil and Recent sample.
A. Continuous Variation
In the quantification of continuous variation, a basic and serious problem is that of how to eliminate various influences caused by the age heterogeneity. Needless to say, all the samples representing local populations consist of individuals of different average size, and none of the samples show a definite pattern of size distribution. Furthermore, in dead shells and fossils the size distribution may be greatly influenced by differential sorting during post-mortem transportation and deposition. Various biases in shell size are also inevitable during sampling in the field and preparation in the laboratory. For example, the representation of small-sized individuals is inevitably influenced by the
mesh size of dredge nets, which is not necessarily uniform in the present samples. The form ratio of height/length (or any other combination of two linear measurements), which has been traditionally used as a morphological characteristic in taxonomy and other comparative studies, actually shifts to some extent with growth. Consequently, meaningful comparison between local populations is not necessarily possible on the basis of such growth-variant characters. Generally speaking, a flat-topped histogram would be obtained, if variation were examined on a shifting morphometric character like this.
As was theoretically and empirically discussed by Imbrie (1956) and Hayami and Matsukuma (1970, 1971), this problem can be overcome, though not completely, by some bivariate analysis with consideration of relative growth. Since the classical works of Huxley (1924, 1932) and Nomura (1926), the relative growth of an organism has been commonly expressed by a power function, y=bxa or log y=a log x+log b, where x and y are two variables of linear measurements, and a and b are called growth ratio and initial growth index, respectively. As was discussed in detail by Gould (1966), the theoretical basis of the power function, as of any function, bears some weakness, but at least empiri-cally the relation between two linear measurements seems to fit well with this function in many organisms. Practically, an equation of average relative growth can be obtained by the logarithmic transformation of measurements and the regression of a best-fit line. As recommended by some authors (Kermack and Haldane, 1950; Imbrie, 1956; Gould, 1966; Hayami and Matsukuma, 1970, 1971), the method of reduced major axis may be most adequate and convenient to determine the relation between two linear measurements which are independent of each other. Individual variation is then expressed by the dispersal around the reduced major axis on the double logarithmic scatter diagram.
If the relative growth of an organism can be roughly expressed by a single allometric equation, in other words, regarded as monophasic allometry, the variability of a bivariate character appears to be indicated by the correlation coefficient (r) between the logarithm of two variables (log x and log y). However, this coefficient is also influenced by the size composition of a sample, especially by the range of each variable. Generally speaking, its value tends to decrease if the sample consists of individuals of similar size. Therefore, the variability of different samples cannot be compared with correlation coefficients, but that of different bivariate characters in one and the same sample can.
In order to analyze the individual variation and relative growth in selected samples of Cryptopecten, the following variables were measured or counted (Fig. 8):
L: Length of valve measured in parallel to hinge axis.
H: Height of valve measured vertically to hinge axis (excluding protruding part above hinge margin).
T: Thickness of odd (right or left) valve measured vertically to commissure plane.
C: Total thickness of conjoined valves (in closed condition) measured vertically to commissure plane.
D: Length of dorsal margin of anterior and posterior wings.
H1: Height from origin of growth to first growth ring.
W: Maximum wave height of commissure in ventral view.
X: Number of radial ribs on disk counted at middle growth stage (7 mm or 10 mm in height).
The adequacy of applying a power function, y=bxa, was ascertained by plotting the bivariate data on double logarithmic papers (some examples shown in Figs. 9, 10, 13).
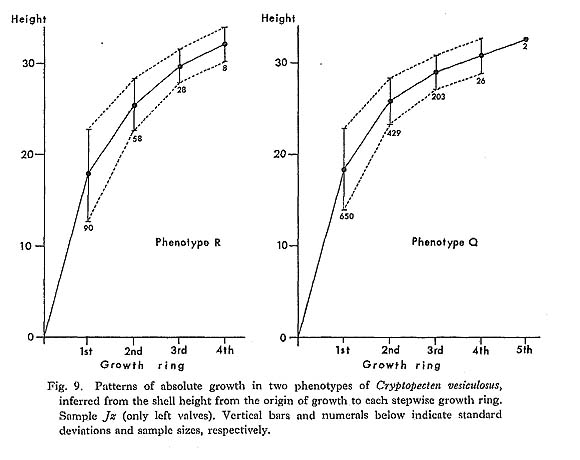
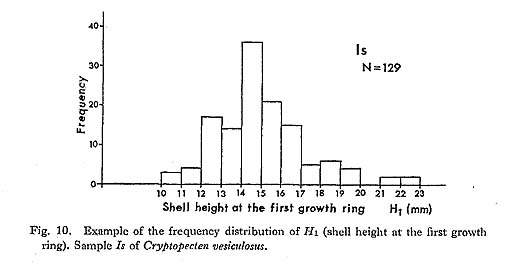
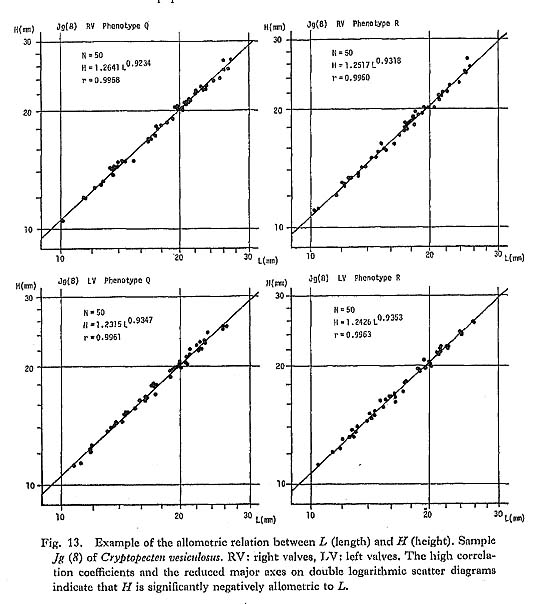
The result of bivariate analysis is shown in Tables 4-10, where the following abbrevia-tions are used:
N: Number of examined individuals
r: Correlation coefficient between'two variables
a: Growth ratio (slope of reduced major axis in double logarithmic scatter diagram), which is obtained by a=slog y/Slog x [s: standard deviation]
: Standard error of growth ratio, which is obtained by 
b: Initial growth index (anti-logarithm of y-intercept of reduced major axis in double logarithmic scatter diagram), which is obtained by log b=log y-a log x
RG: Mode of relative growth
I: "Isometry" (null hypothesis of a=1 not rejected with 95 percent confidence)
NA: Negative allometry (a significantly smaller than 1 with 95 percent confidence)
PA: Positive allometry (a significantly larger than 1 with 95 percent confidence)

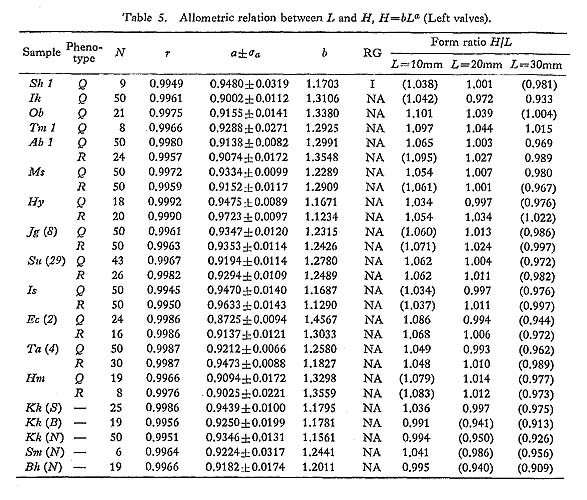


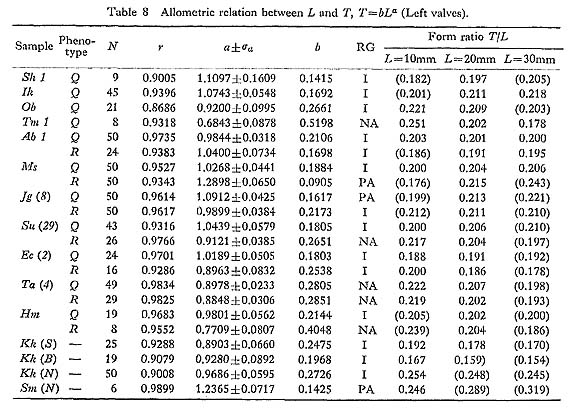

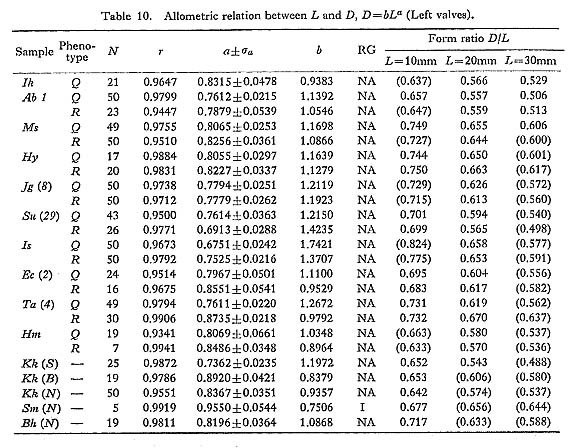
In the same tables three values of form ratios are also indicated. They were theoretically obtained from each allometric equation at three fixed sizes (L=10 mm, 20 mm and 30 mm). The values in parentheses indicate that the observed range of L does not cover the fixed size.
Generally speaking, comparison of the growth ratios (a) of allometric equations seems
to be meaningful, but that of the initial growth indices (b) does not because the latter means the value of at x=1 and is clearly dependent on a (see Imbrie, 1956, for the statistical comparison of a bivariate character between two samples). The form ratios at fixed sizes are probably useful for comparative studies, because the influence of size difference between samples is largely eliminated.
1. Shell Size and Growth Rate [Table 3]

Shell size is undoubtedly one of the most easily measurable univariate characters, variation being controlled primarily by polygenic factors. The simple overall size of shell is, however, not very useful, unless the influence of age heterogeneity is eliminated by some adequate method. In this respect, the partial height of shell from the origin of growth (or hinge-axis) to the first stepwise growth ring, here denned as H1, may be more significant, because it seems to indicate the growth amount from fertilization to the first unfavorable season for growth. Judging from the data on living populations in the eastern part of Sagami Bay, this value directly indicates size at attainment of sexual maturity. Annual growth amount of an individual seems to be shown by the interval of successive growth rings, each of which is laid in relation to the annual reproductive season.
The intrapopulational variation of H1 is quite wide in every sample, the coefficient of variation (V) commonly exceeding 15 (Table 13). As exemplified by sample Jz (Table 3, Fig. 9), however, the variation generally becomes much narrower at the subsequent ring(s). The reason why the size variation decreases with growth must be further investi-gated, but it is, I think, mainly clue to the long duration of breeding season. If fertilization occurred for several successive months every year, the reduction of variation would be logically explicable, because the influence of age heterogeneity and the absolute growth rate are generally much reduced with growth.
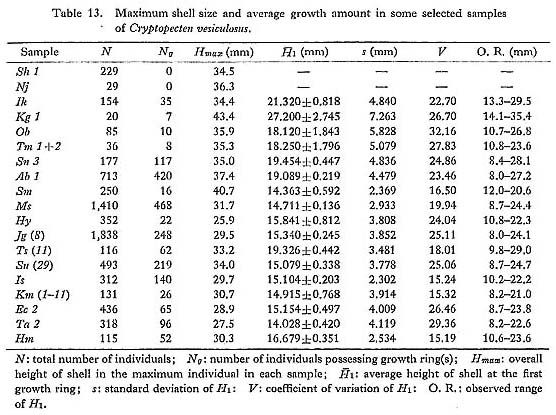
In most Recent samples the frequency distribution of H1 is unimodal, the mode lying at about 15 mm (Figs. 10 and 11). In some Late Pleistocene samples, however, the distribution is clearly bimodal. In the fossil sample Jz, for example, there are two clusters in the histogram of H1; the mode of the smaller cluster lies at about 10 mm and that of the larger one is near 21 mm (Fig. 12).
Bimodal distribution of H1 may in fact be due to the occurrence of two breeding seasons a year, as is the case with Pecten (Pecten) maximus in the Irish Sea (Mason, 1957). In the present case, however, I presume for the following reasons that the first ring in the smaller cluster is largely unrelated to reproductive activity.


1) The first ring in the smaller cluster is generally, if not discriminately, weaker and less stepwise in comparison with subsequent rings.
2) The growth rate and ultimate shell size in these Late Pleistocene samples are
much larger than those of Recent ones. Therefore it is rather unlikely that individuals reach sexual maturity at such a small size.
3) The average shell size of the smaller cluster at the next (second) ring is not much different from that of the larger cluster at the first ring (Fig. 12).
If this interpretation is correct some amendments may be necessary as to the numbering of growth rings and statistical treatment of shell size. The average of H1 would then becomes considerably larger, and its standard deviation somewhat smaller. Furthermore, not only in the bimodal samples but also in apparently unimodal samples, it is by no means proven that the first ring at very small size (less than 10 mm) indicates sexual maturity. It can be said, however, that this ambiguous ring occurs only in a small fraction of each population sample. In other words, even if the numbering of rings were partly wrong, this would not seriously influence the discussion and conclusion at this level.
2. Length [L] versus Height [H] [Tables 4 and 5]
The simple ratio of H/L appears to indicate the degree of vertical elongation of disk.
In almost all the samples of C. vesiculosus this ratio may or may not exceed 1, and varies considerably among individuals. The frequency distribution of H/L, as well as other form ratios, is commonly more or less flat-topped (platykurtic).
For instance, various basic statistics of this character calculated on 100 individuals randomly selected from the sample Is are as follows:
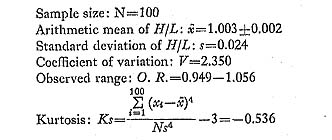
The kurtosis is an interesting index. As was clearly explained by Simpson, Roe and Lewontin (1960), a negative (or positive) value of Ks indicates that the frequency distribu-tion is platykurtic (or leptokurtic) in comparison with ideal normal distribution. The
negative value of Ks in this case is rather remarkable, indicating a considerably flat-topped distribution. This pattern is perhaps largely due to the age heterogeneity, because the average value of H/L actually becomes much smaller with increase of shell size, as shown below:

The average relative growth between length (L) and height (H) was examined on 44 lots of 13 selected samples of C. vesiculosus by the method of reduced major axis. In all of these samples, both in right and left valves, log L shows extremely high correlation with log H; the correlation coefficient (r) exceeds 0.99 except for one small sample. As an example, the L-H relation in subsample Jg (8) is shown in Fig. 13. The growth ratio (a) varies considerably with the sample, but in almost all lots the values are significantly smaller than 1, and the relative growth is regarded as negatively allometric with 95 percent confidence (see Hayami and Matsukuma, 1970, 1971, for the statistical test for discrimi-nation between "isometry" and allometry). The obtained values of a are seldom signif-icantly different between two valves and between two phenotypes within one and the same sample, though they sometimes differ between different samples.
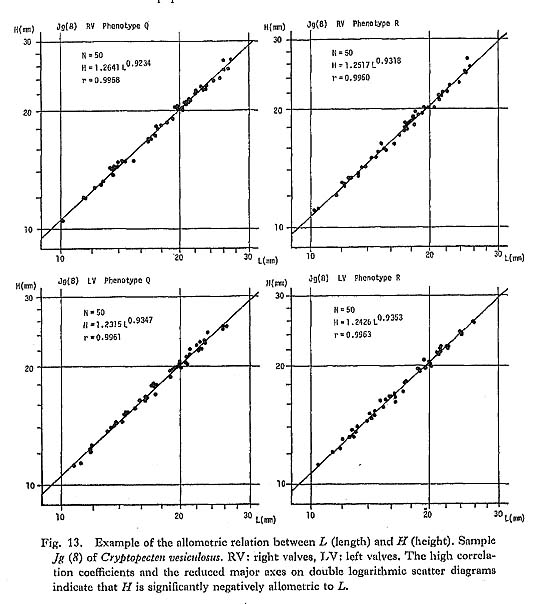
C. spinosus sp. nov., which is proposed in this article on fossil sample Kk (S), seems to exhibit the same tendency with respect to this bivariate character as C. vesiculosus.
In C. bullatus, though the sample size and number of samples are small, the ratio of H/L is generally smaller than in C. vesiculosus at every fixed size. So far as samples Hs (B) and Kk (B) were examined, negatively allometric growth is also apparent.
In C. nux only two fossil samples, Kk (N) and Sm (N), and one Recent sample, Bh (N), were morphometrically analyzed, other samples being too small. In sample Kk (N) the ratio of H/L is evidently smaller than that of C. vesiculosus and C. spinosus at every growth stage and nearly comparable with that of C. bullatus. Negatively allometric growth is also recognized in each valve. On the other hand, the right valves of Sm (N) show "iso-metric" growth in this character, though this may be due to the relatively small sample size.
3. Length [L] versus Thickness [T] [Tables 6 and 7]
The shell of Cryptopecten is highly inequivalve in every species. Except for the early stages of growth, the right valve is more strongly convex than the left, and the mode of relative growth of the two valves is quite different. The present fossil and dead shell samples are mostly composed of odd valves, and the bivariate analysis on the L-T relation was independently carried out for right and left valves. The curvature of shell
surface, as noted before, often changes periodically in accordance with the annual gonad development. Therefore, the shell convexity must be influenced not only by the age composition but also by the season when the individuals died. This is partly responsible, I think, for the relatively low value of correlation coefficient between log L and log T.
The L-T relation for the right valves of C. vesiculosus was examined on 18 lots of 11 samples. In all cases the ratio T/L becomes much larger with growth, even though minor oscillation may occur during the growth of each individual in relation to periodical change of surface convexity. The correlation coefficient between log L and log T is generally higher than 0.95, but always decidedly lower than that between log L and log H. This is evident if the variability in the scatter diagram (Fig. 13) is compared with that of Fig. 14, which is based on the same material. Except for a few small samples, the growth ratio (a) is significantly larger than 1, indicating that T is positively allometric against L. The difference of a is commonly insignificant between the two phenotypes belonging to the same sample, but is sometimes very significant between different samples.
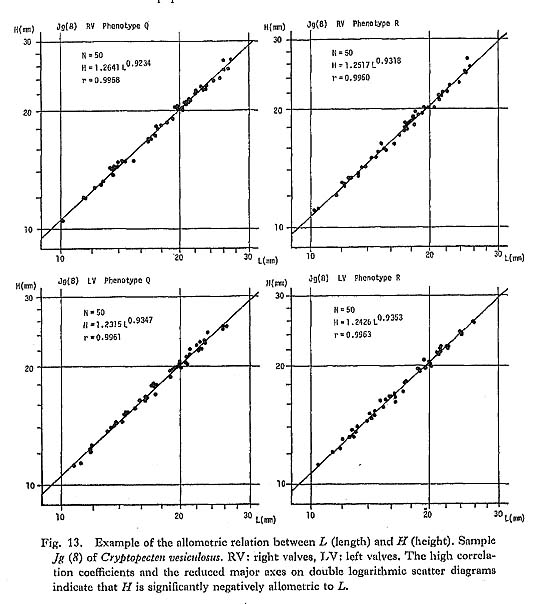
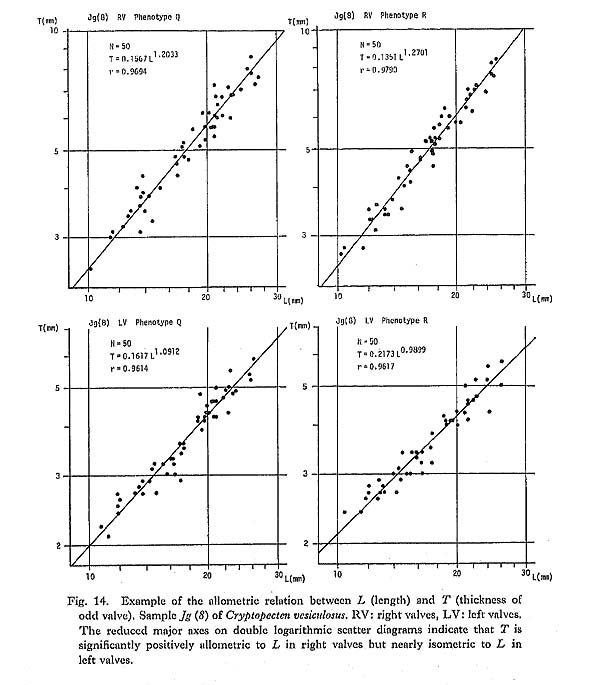
The shell convexity and L-T relation in the left valves were also examined on 18 lots of the same samples of C. vesiculosus. The ratio of T/H is much smaller than that of the right valves after the growth stage of L=10 mm. The correlation coefficient (r) between log L and log T is generally lower than the value for the right valves in each sample. Since the ranges of shell size are not much different for the two valves in each sample, this fact seems to indicate that the shell convexity is commonly more variable in the left valves than in the right. The growth ratio (a) may or may not exceed 1, and varies con-siderably with the sample. Of 18 regressions positive allometry and negative allometry were recognized in two and five lots, respectively, and the other eleven lots are regarded as "isometric".
C. spinosus sp. nov., for which only one fossil sample Kk (S), is available, displays
weaker convexity of the two valves, but does not appear to differ from C. vesiculosus in mode of relative growth.
C. bullatus also shows much weaker convexity of both valves than C. vesiculosus, as is apparent in the ratio T/H at the size of L=10 mm. So far as the sample Kk (B) was examined, the right valves clearly show positive allometry, but the hypothesis of "iso metry" is not rejected in the left valves. In spite of the wide range of shell size in this sample, the correlation coefficient (r) in each valve is considerably low. The variability of this bivariate character is probably somewhat greater in C. bullatus than in C. vesiculosus.
C. nux, on the other hand, is characterized by a stronger convexity of both valves than other species of Cryptopecten. The L-T relation was examined in two fossil samples, Kk (N) and Sm (N). Though the sample size of Sm (N) is not very large, the mode of relative growth appears to be considerably different between the two samples; the L-T relation in each valve is "isometric" in Kk (N) but clearly positively allometric in Sm (N). The ratio of T/L in Sm (N) is also much larger than that in Kk (N) at the same growth stages.
In all of these four species the right valves always have higher values of growth ratio (a) and lower values of initial growth index (6) than the left valves of the same sample. This indicates a left-convex shell in the very early stages and agrees with the result of SEM observation of the umbonal part of shells. In all the samples the reduced major axes of both valves cross with a large angle, and the shell becomes right-convex, as is indicated by the simple ratios T/L. The allometric relation between L and T in these species is collectively shown in Figs. 15 and 16 on the basis of several large samples. Similar patterns of L-T relation may be observed in many species of the Pectinidae (expecially Chlamydinae), but such a strong contrast between the valves is probably rare.

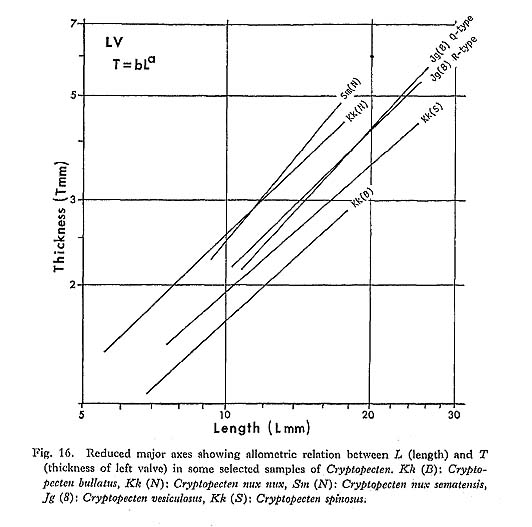
4. Length [L] versus Thickness of Conjoined Valves [C] [Table 8]
Because most samples of living shells are small in size, the relation between length (L) and total thickness (C) was examined on only two samples, Hy and Is, in C. vesiculosus and one sample, Bh (N), in C. nux. In the former species log L and log C show high correlation, and the growth ratio (a) is significantly larger than 1, indicating the relative growth to be positively allometric. This is probably due to the highly positively allo metric growth of the right valve. The values of a are not significantly different for the two phenotypes in each sample. C. nux generally shows stronger convexity of two valves than C. vesiculosus, but, as is intuitively recognized in Bh (N) and many other samples, the intrapopulational variation of C/L appears to be much greater than that of C. vesi culosus. Bivariate analysis on Bh (N) resulted in rather low correlation between log L and log C, and null hypothesis of "isometry" is not rejected. It may be, however, partly due to the small sample size.
5. Length [L] versus Dorsal Length of Wings [D] [Tables 9 and 10]
The relation between the overall length (L) and the length of dorsal margin of the two wings (D) was examined on 39 lots of 12 samples of C. vesiculosus. These samples are essentially the same as those used in other bivariate analyses, but several individuals were excluded because of broken ends of anterior and/or posterior wings.
The ratio D/L actually seems to indicate the size relation between the disk and the wings. As is indicated by the values of D/L at three fixed sizes, the ratio becomes much smaller with growth. In other words, the auricular part is relatively large in early stages but becomes smaller with growth. This tendency may be ubiquitous in many species of the Pectinidae, but the allometric growth is quite remarkable in this case.
As a result of bivariate analysis, it was found that the correlation coefficient (Υ) between log L and log D is generally higher than 0.95, and the growth ratio (a) is almost always significantly smaller than 1 with 95 percent confidence, indicating that relative growth is negatively allometric in each valve. Though there are a few exceptions, the values of a are not significantly different for the two phenotypes and nor for the left and right valves in one and the same sample. Fig. 17 is an example of this bivariate analysis.
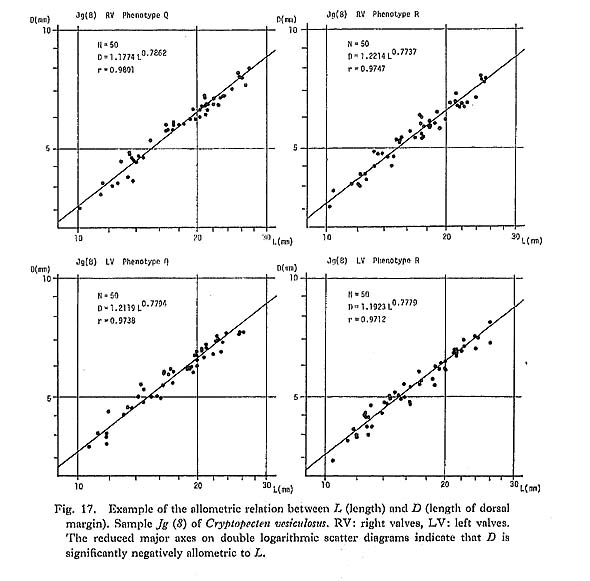
Meaningful bivariate analysis of L-D relation is now difficult on the Recent samples of C. bullatus and C. mux, because the sample size is always too small. However, if the individuals belonging to different samples are collectively examined, the auricular part seems to become proportionally smaller in these species as well. Examination of the fossil samples, Kk (S), Kk (5) and Kk (N), showed that the growth ratio (a) is significantly smaller than 1 in C. spinosus, C. bullatus and C. nux, indicating evident negative allometry. Therefore, it is concluded that the L-D relation is essentially similar in these four species of Cryptopecten.
6. Number of Radial Ribs [X] [Table 11]
The number of radial ribs on the disk is a univariate character currently applied for the identification and classification of pectinids. In every species of Cryptopecten, radial ribs are quite regular in prominence, neither bifurcated nor, except for very early dissoconch, inserted during the course of growth. Their number would therefore appear to be a growth-invariant character. As noted above (p. 22) however, in many individuals the
outermost radial ribs near the anterodorsal and posterodorsal margins of disk are much weakened or have vanished altogether in the course of growth. I thus define X to be the number of radial ribs counted at the middle stage of growth (approximately 10 mm in height in C. vesiculosus, C. bullatus and C. yanagawaensis; approximately 7 mm in C. spinosus and C. nux).
This character was examined on 135 lots of 22 samples of C. vesiculosus, three lots of one sample of C. spinosus, six lots of two samples of C. bullatus, six lots of two samples of C. nux, and three lots of one sample of C. yanagawaensis. The results are collectively given in Table 11, where the mean
, standard error
, standard deviation (s), coefficient of variation (V), observed range (O. R) and mode of frequency distribution
are indicated.
In C. vesiculosus the mode commonly lies at 14 or 15 in Recent and younger fossil samples, but at 16 in such older fossil samples such as Ik and Kg 1. The histograms arc almost always unimodal and resemble normal frequency distributions. The uniformity of variability of this character is worthy of notice; the standard deviation (s) is almost always confined to the range from 3.0 to 6.0. T-tests show that the values of
for each of the two valves in the same sample are seldom significantly different. The same is prob ably true for the two phenotypes, though in many cases Phenotype R seems to show a slightly smaller value of
than Phenotype Q. This is probably because the radial ribs of Phenotype R are weaker and less conspicuous, especially near the peripheries of disk, than those of Phenotype Q.
One of the distinguishing characters of C. spinosus is, as shown in the sample Kk (S), the significantly smaller number of radial ribs in comparison with the other species of Cryptopecten; the number ranges from 12 to 15, and the mode lies at 14.
C. bullatus has, meanwhile, more numerous radial ribs than C. vesiculosus. Though every Recent sample consists of a small number of individuals, the values of
are ap proximately 20 in the samples USNM 764152 and Hs (B). This is not much different from the value of the Hawaiian and Philippine specimens including the holotype of C. alii. The fossil sample Kk (B), which is identical with C. bullatus in various other characters, nevertheless shows a somewhat larger number of radial ribs.
C. nux also possesses more numerous radial ribs than C. vesiculosus. Most specimens of the fossil samples Kk (N) and Sm (N) as well as several Recent and fossil specimens from various localities have 18 to 22 radial ribs.
C. yanagawaensis seems to have the most numerous radial ribs of the five species of Cryptopecten. In the solitary fossil sample Mn (Y) most specimens possess 20 to 25 radial ribs with the mode lying at 21 or 22. The variability of this character may not be so great as was suggested by Masuda (1958).
Although it is unknown whether this character is entirely controlled by genetic factors or not, I conclude that the number of radial ribs of Cryptopecten, is almost entirely growth- invariant and can be regarded as a useful univariate meristic character for discussions of geographic variation and evolutionary change as well as specific distinction.
B. Discontinuous Variation
Discontinuous variation is a phenomenon which is characterized by the presence of
two or more discrete phenotypes within an interbreeding population. It may or may not be controlled by genetic factors. The term "polymorphism" is sometimes used for any kind of discontinuous variation, but, as was discussed by Mayr (1963, p. 150), such nongenetic discontinuous variations as age, social, ecological and traumatic variations are preferably distinguished from true polymorphism and treated under a different term, polyphenism. Ford (1940, 1964) denned genetic polymorphism as "the occurrence to gether in the same locality of two or more discontinuous forms of a species in such pro portion that the rarest of them cannot be maintained by recurrent mutation".
Most genetic approaches to evolution at the morphological level have been carried out on the basis of polymorphism, simply because the gene frequency of populations and its change in time and space can be easily recognized phenotypically. In marine organisms the genetic background of discontinuous variation is often difficult to determine. Still, discrimination between polymorphism and polyphenism is possible through careful observations of morphology, population structure and ecological habit as well as through analogy with well-experimented organisms.
1. Color Polymorphism
Polymorphism of shell coloration seems to be widespread in molluscs. In certain groups of the Bivalvia, ground color and color pattern vary discontinuously within one and the same population. Discontinuous variation of ground color is also quite striking in several species of Chlamys, Aequipecten, and some other genera of the Pectinidae. In the beautiful Japanese species, Chlamys (Mimachlamys) nobilis (Reeve), for example, most individuals are reddish brown in color, but orange, yellow, purple, and white individuals are occasionally found together in the same population. So far as I am aware, no Mendelian experiment has been successfully carried out, but the color variation of pectinids is probably genetic. In some land snails polymorphism in ground coloration and color banding is controlled by a series of multiple alleles constituting a supergene (Cain and Sheppard, 1950; Komai and Emura, 1955; etc.).
In the four extant species of Cryptopecten, most individuals are reddish-brown to magenta in ground color, frequently mottled with irregular oblique bands or spots of pale coloration. Certain individuals (less than 5 percent in C. vesiculosus) are yellow or orange in ground color. Though yellow and orange individuals are sometimes not clearly sepa rable from each other, they are never mottled but quite monotonic in coloration. The phenotypic frequency of yellow plus orange individuals can thus be clearly recognized in Recent samples, and it would be useful for the analysis of geographic variation if very large fresh samples could be examined. These features of color polymorphism are es sentially similar in C. vesiculosus, C. bullatus, C. nux and C. phrygium, suggesting that these species are closely related to one another and have a common ancestry.
Color polymorphism of Cryptopecten is an interesting phenomenon but generally difficult to observe in fossil samples. Mottled color pattern of Late Pleistocene fossil specimens are often visible (generally more clearly under ultraviolet light), but variation of ground color is hard to recognize.
2. Dimorphism of Surface Sculpture in C. vesiculosus [Table 12]
As pointed out previously (Hayami, 1972, 1973), every sample of C. vesiculosus after the Middle Pleistocene consists of two discrete phenotypes, called Q and R, without any intermediate individual. The morphological difference between these becomes clear after the beginning of the third stage of dissoconch. The fundamental radial rib structure, consisting of a central solid ridge and lateral hollow parts, is the same for the two phenotypes, but in transverse section the radial ribs of Phenotype Q are clearly more quadrate and highly elevated than those of Phenotype R. This difference is well reflected in the ratio between the overall height (H) and the maximum wave height of the com missure (W) in ventral view as studied previously on the samples Is and Jz (in part) (Hayami, 1973, figs. 4 and 5: here reproduced in Figs. 18 and 19). Clearly bimodal histograms of the ratio W/H were obtained and the two clusters correspond well with the two phenotypes.
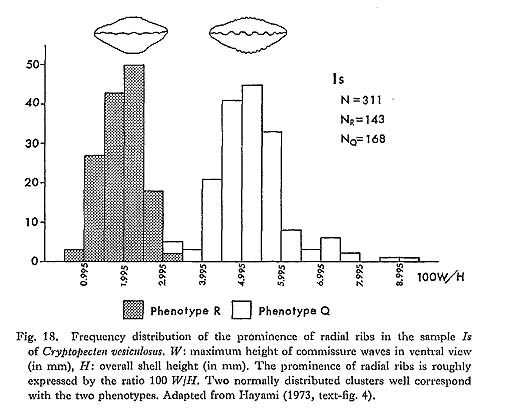

A more conspicuous difference between the two phenotypes exists in the sculpture on the interspaces of radial ribs, especially in the inclination of scales and the shape of hollow parts, as will be described in detail in the systematic description (p. 23). The scales covering the hollow parts in both phenotypes are frequently exfoliated in abraded dead shells and fossils. Nevertheless, the distinction between the two phenotypes is always quite clear and never arbitrary. Therefore the phenotypic frequency can be pre cisely determined in every sample. The results are collectively shown in Table 12.
The individuals belonging to Phenotype Q seem to be further separable into two sub phenotypes by the presence or absence of erect scales on the top of the central ridges (see Plate 4, Figs. 1, 2, Plate 5, Figs. 1, 2). The two subphenotypes, called rough type and
smooth type, are discontinuous with few intermediate individuals. For example, among 169 individuals belonging to Phenotype Q of the sample Is, 60 and 109 individuals are assigned to the rough type and smooth type, respectively. The frequency of the two sub phenotypes is also recognizable, though somewhat arbitrarily, in some well-preserved dead shell and fossil samples (Plate 6, Figs. 1, 2), but their discrimination is generally difficult in the case of abraded specimens and individuals smaller than 10 mm in length. The top of radial ribs in Phenotype R is, on the contrary, always quite smooth, lacking any erect scales. This fact may suggest that the discontinuous variation between the two subphenotypes is controlled by some cause related to the factor determining the pheno typic difference. Although the smooth subphenotype appears at a glance to be morpholog ically intermediate between the rough subphenotype and Phenotype R, the hypothesis of Hardy-Weinberg equilibrium, that the first were heterozygote individuals between two homozygote ones, is statistically rejected in Is and some other large samples.

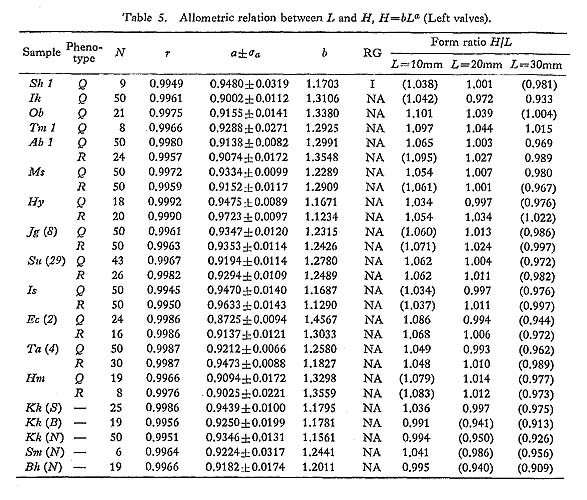

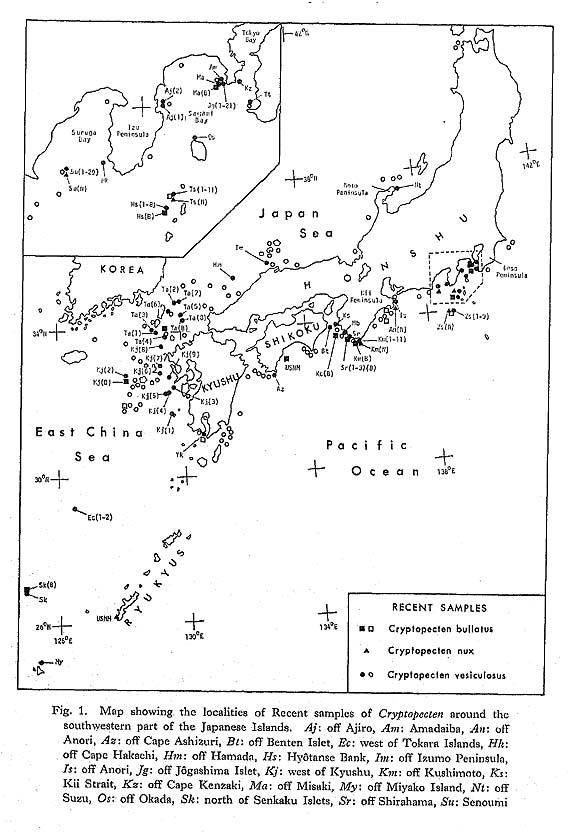
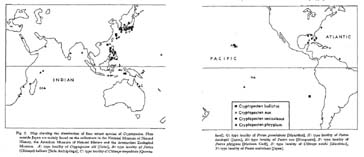
Fig. 2. Map showing the distribution of four extant species of Cryptopecten. Plots outside Japan are mainly based on the collections in the National Museum of Natural History, the American Museum of Natural History and the Amsterdam Zoological Museum. A: type locality of Cryptopecten alli [Oahu], B: type locality of Pecten (Chlamys) bullatus [Sulu Archipelago], C: type locality of Chlamys corymbiata [Queens-land], G: type locality of Pecten guendolenae [Mauritius], H: type locality of Pecten hastingsii [Japan], N: type locality of Pecten nux [Marquesas], P: type locality of Pecten phrygium [Mexican Gulf], S: type locality of Chlamys smithi [Mauritius], V: type locality of Pecten vesiculosus [Japan].
|
Judging from the following facts, these phenotypes are taxonomically inseparable in spite of the clear morphological discontinuity.
1) Living individuals belonging to the two phenotypes were always found together within the same dredge sample (or subsample) (e. g., Kz, Jg (1-9, 16-23), Zs (4, 8), Hk) (Table 1).
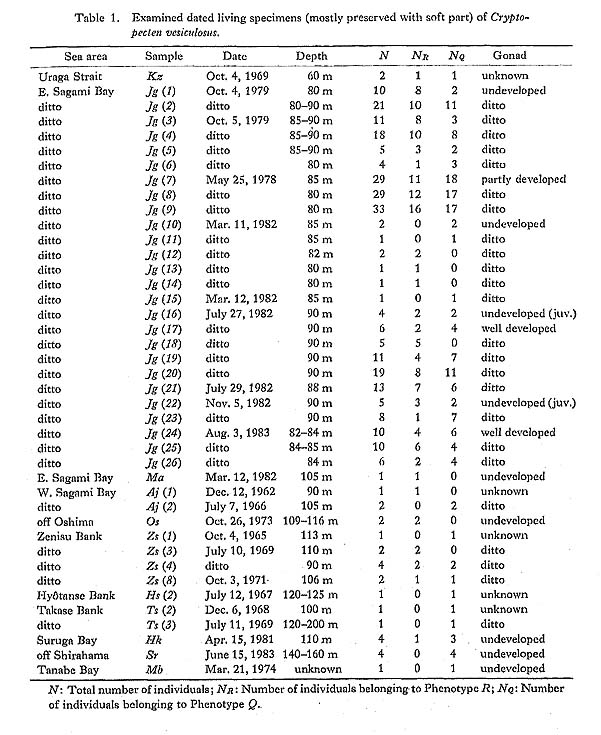
2) The two phenotypes are coexistent in all the large samples of Recent dead shells as well as fossils after Middle Pleistocene. The phenotypic frequency is considerably stable in the same sea area, though some geographic variation and chronological change
are known, as discussed later. Perfect distribution overlap of this sort is quite unlikely in the case of sibling species.
3) The two phenotypes are clearly distinguishable by their different sculpture, but no significant difference can be observed as to their coloration, size, growth rate presumed from the intervals of growth rings, average number of radial ribs, various bivariate characters of shell, and anatomical characters of soft parts. This dimorphic phenomenon is therefore quite independent of the variation of other characters.
4) As a result of my examination of living populations in Sagami Bay, it was found that the breeding season is not different for the two phenotypes. The reproductive glands are fully developed in all the large specimens of both phenotypes, which were collected near the end of July (subsamples Jg (16-21)) and the beginning of August (subsamples Jg (24-26)).
Though nobody has successfully observed any living population of C. vesiculosus in its habitat, the ecological habits of the two phenotypes seem to be the same, judging from my own observation on freshly dredged specimens and their behavior in aquaria. As already described, this pectinid is certainly hermaphroditic, and this discontinuous variation is never attributable to sexual dimorphism.
Such discontinuous variation of sculpture is unknown in other species of Cryptopecten. All the available samples of C. bullatus, C. nux, C. phrygium, C. spinosus and C. yanagawa ensis, as well as those of C. vesiculosus predating the Middle Pleistocene, are monomorphic in this character, always exhibiting erect (not imbricated) scales on the interspaces of radial ribs like the Phenotype Q of C. vesiculosus. In this respect Phenotype R seems to represent a more specialized and probably more advanced morphology than Phenotype Q, and I regard here the former as mutant and the latter as wild-type.
Somewhat similar dimorphic phenomena can be observed in some other pectinids. As will be shown later, the populations of Aequipecten commutatus (Monterosato, 1875) from the Mediterranean seem to consist of two discrete phenotypes, the radial ribs of which may or may not have a hollow structure. In Aequipecten opercularis the surface of radial ribs and interspaces is either rough or smooth as with the two sub phenotypes in Q-type individuals of C. vesiculosus.
The relation between Volachlamys hirasei (Bavay, 1904) and Volachlamys awajiensis (Pilsbry, 1905) is another striking case which I have observed. The surface sculpture of the two nominal species appears to be quite different; the radial ribs are rounded and much weakened in V. hirasei, whereas they are subquadrate and highly elevated in V. awajiensis. For a long time many Japanese malacologists have regarded the two phena as specifically or subspecifically distinct, but the latter was sometimes regarded as a variety or a forma of the former (Kuroda, 1932; Habe, 1951). Though individual varia tion has been little studied at the population level, the two phena are distributed in the same sea areas (at least in the Inland Sea between Honshu and Shikoku, and Ariake Bay
of Kyushu), and are hardly distinguishable by such characters as shell size, coloration, general outline and number of radials. As a result of my preliminary survey on the collec tion of the National Science Museum [Tokyo], it was found that the sample NSMT 46502 labelled V. hirasei awajiensis and the sample NSMT 46503 labelled V. hirasei hirasei came from one and the same locality (Okinohata Harbor of Yanagawa City, Kyushu). Although there are a few intermediate individuals, they are basically discontin uous. Nevertheless the two samples probably came from one and the same local popula tion. According to Dr. S. Okamura's personal communication (May 15, 1982), the two phena are also distinct but strictly sympatric at several stations in Osaka Bay. At present I am strongly inclined to regard the relation of the two phena as an example of intra populational discontinuous variation which is somewhat analogous to the case of C. vesiculosus. If this is true, V. awajiensis should be taxonomically treated as a junior synonym of V. hirasei, merely representing a sharply ribbed phenotype within one and the same species.
C. Evaluation of Ecophenotypic Effect
Of various types of ecological variation enumerated by Mayr (1969, pp. 152-155), habitat variation (or ecophenotypic effect) must be critically examined in studies of morphological variability. This effect may be defined as a purely nongenetic modification (or adjustment) of morphology in response to specific ecological conditions.
Among the various bivalves, ecophonotypic variation including xenomorphism (Stenzel, 1971, pp. N1021-1023) is predominantly known in the Ostreidae and some other groups whose shells tightly attach to hard substrates. Such ecological variation, though with less conspicuous effect, may also exist in byssate and free-living species. According to Beu (1966), for example, there are two ecophenotypes in Chlamys dieffenbachi (Reeve) from the southwestern Pacific; one is encrusted by sponge and the other is free-living. In this pectinid the number of radial ribs increases with growth, the increase occurring ontogenetically earlier and the shell growing much larger in the sponge-encrusted indi viduals than in the free-living ones. Consequently, it may be said that the number of radials varies ecophenotypically. Johnson (1981) interpreted the wide intrapopulational variation and ontogenetical flexibility of this character in the Jurassic species, Radulopecten vagans (Sowerby), as due to some ecophenotypic effect on the basis of analogy with the case of C. dieffenbachi, predicting that ecophenotypically varying organisms may be generally characterized by an ontogenetic reduction in variation.
So far as the number and mode of radial ribs of Cryptofecten are concerned, however, an ecophenotypic effect of this sort is unlikely in view of the following facts:
1) The radial ribs in every species of Cryptopecten are quite persistent except for the outermost ones, and their number never increases with growth. The variation is not flexible and seems to be inherently determined.
2) In each sample the frequency distribution of rib number is unimodal, resembling a normal curve.
3) Judging from the dredged living shells of C. vesiculosus in Sagami Bay, there is no evidence for ecological seqregation of the two phenotypes; their mode of life as well as attached organisms seem to be quite the same.
4) The phenotypic frequency is almost stable in the same sea area.
For the same reasons, I believe that other non-genetic causes (e. g., climatic, host determined, density-dependent and traumatic) are improbable for the sculpture di morphism of C. vesiculosus.